Alkali-silica reactivity (ASR), a pervasive durability problem that
occurs in portland cement concrete, is responsible for the premature
deterioration of various types of concrete structures in the United States
and around the world. While lithium compounds have been recognized for more
than 50 years as being effective in preventing concrete expansion due to
ASR, there has been increased interest in recent years in using them to both
treat existing structures and as a preventive measure in new concrete
construction. |
The best way to avoid ASR in new concrete is to take
precautions in the mix design. These include testing aggregates for
reactivity; use of low-alkali cements, suitable pozzolans like ASTM C-618
Class F fly ash, and lithium-based admixtures. |
How lithium inhibits ASR?
Lithium nitrate reaction with reactive silica
and moisture is similar to other alkalies such as sodium and
potassium. Unlike ASR, however, the gel formed by Lithium Nitrate with alkalis
does not absorb excess moisture, preventing harmful expansion. |
From the industry's point-of-view, use of an ASR
inhibitor not only better serves clients, but also can protect from
potential legal action that could arise if ASR is not mitigated when
state-of-the-art remedies are available. |
The cracks radiate from the interior
of the aggregate out into the surrounding paste. The cracks are empty (not gel-filled) when formed. Small or large amounts
of gel may subsequently exude into the cracks. Formation of the alkali silica gel does
not cause expansion of the aggregate by itself. Observation of gel in concrete is
therefore no indication that the aggregate or concrete will crack. Only
moisture can cause the expansion and resultant cracking Alkali silica reaction is diagnosed primarily by four main
features
|
From this brief overview of the literature on the mechanisms, the
following
summarizes present knowledge on ASR:
- ASR is a reaction between the OH in the pore solution with amorphous
or poorly
crystallized silica in the aggregates.
- The reaction product imbibes water and expands.
- The presence of water or RH higher than 80’% is necessary for the
gel formed to
expand and induce concrete cracking.
- Some siliceous mineral admixtures deplete the alkalis horn the pore
solution, lowering
the pH, therefore decreasing the likelihood of ASR.
- The aggregate type and size distribution play a significant role in
the expansion
measured in concretes
- Other factors influencing the cracking due to ASR include air
entrainment and possibly
WlC.
|
ASR-induced cracking can be confused with other forms of
cracking. As a result, inspectors sometimes misdiagnose the problem and then
apply rehabilitation techniques that may actually make the ASR problem
worse. Inspectors and engineers therefore need better, more accurate tools
for identifying ASR in existing concrete structures and for deciding on the
best treatment for existing and current structures. Several such tools were
developed under the Strategic Highway Research Program (SHRP): • Handbook for the Identification of Alkali-Silica
Reactivity in Highway Structures, an easy-to-use field guide for
distinguishing ASR-related cracking in various types of concrete roads and
structures.
• A fast and simple test for detecting the presence of
ASR in concrete.
• A fast and reliable means of determining an
aggregate’s potential reactivity to alkalis.
• Eliminating or Minimizing Alkali-Silica
Reactivity, a report that identifies available options for alleviating
ASR damage in concrete pavement and structures. |
ASR expansion can be reduced to acceptable levels by use of Type F fly
ash and by use of lithium nitrate additive in accordance with the
manufacturer’s recommendations. |
The only indisputable evidence that ASR has developed in concrete is
the presence of ASR gel reaction products. In the early stages of
reactivity, or under conditions where only small quantities are produced,
ASR gel is virtually undetectable by the unaided eye, and revealed only with
difficulty by a skillful observer using a microscope. Thus, ASR may go
unrecognized in field structures for some period of time, possibly years,
before associated severe distress develops to force its recognition and
structure rehabilitation. Use of uranyl (uranium) acetate fluorescence
method has been developed. This method can be used to monitor possible ASR
prior to development of serious distress and to confirm ASR existence. ASR
is uniquely characterized by production of a gel-like reaction product. It
is composed of essentially of silica, the alkalis (sodium and potassium),
and calcium in the presence of water. Uptake of water by the gel is the
primary factor determining volume changes associated with ASR. The gel may
be present in large or minute amounts in aggregates, aggregate sockets, air
voids, fractures, and on the surfaces of externally formed concretes. By
application of uranyl acetate solution to a surface containing the gel, the
uranyl ion substitutes for alkali in the gel, thereby imparting a
characteristic yellowish-green glow when viewed in the dark using short
wavelength (254 nanometer) ultraviolet light. ASR gel fluoresces much more
brightly than the cement paste due to the greater concentration of alkali
and, therefore, the uranyl ion in the gel.
The presence of ASR gel will be revealed in UV light by a yellowish-green
fluorescent glow. Deposits will be localized in cracks, air voids, certain
aggregate particles and, in severe cases, as broad films in aggregate
particles and fractured surfaces. Such films on sawed and cored surfaces may
reflect as "smear" from sawing or cutting. Fractured surfaces eliminate this
effect and most clearly reveal undisturbed ASR gel deposits.
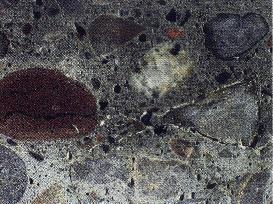 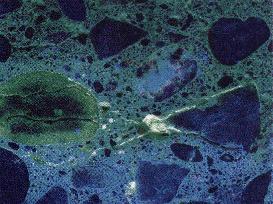
|
Damage due to alkali-silica reaction (ASR) in concrete is a phenomenon
that was first recognized in the U.S. since 1940 and has since been observed
in many countries. Despite numerous studies published, the mechanism is not
yet clearly understood. Nevertheless, the three major factors in concrete
have been identified, i.e., the alkalies contained in the pore solution,
reactive amorphous or poorly crystallized silica present in certain
aggregates, and water. It was found that air content is the most important
variable (other than the three majors factors cited above) that increase
expansion of concretes affected by ASR. |
2.1. ASR Mechanisms
Most researchers agree that the main reaction of ASR is the reaction between
certain forms of silica present in the aggregates and the hydroxide ions (
OH) in the pore
water of a concrete. Very early in the hydration of cement calcium
ions are
incorporated in the hydration products but potassium and sodium stay in
solution and
eventually they are partially incorporated into calcium silicate hydrate
(C-S-H) and
monosulfate (AFJ). Hydroxide ions from the hydration of portland cement
result in a pore
solution having a pH of at least 12.5. Soluble alkalies raise the pH to
about 13 or
higher. Also, the amount of alkalis present in the pore water is related to
the amount of
soluble alkalis present in the cement. If the
silica is well crystallized the vulnerable sites are only at the exterior
surface of the
aggregate (Figure 1a), but in the case of poorly crystallized silica, there
are many
vulnerable sites in the aggregate structure, leading to disintegration of
the silicate network. To keep a neutral charge balance, the cations Na+ and
K+ diffuse
toward the hydroxide ions to react with them and the resulting product is a
gel-like material. The migration of cations of
Na+ and K+ is
slow, therefore the migration of Ca2+ takes place. If the gel is high in
calcium then the gel
is not expansive when exposed to water and, therefore, may not induce
cracking in
concrete. This theory rests on the assumption that calcium could be
available. Diamond
found that there is very little calcium in the pore solution.
This is expected since
the high pH causes the volubility of Ca(OH)2 to be depressed. Nevertheless,
calcium could
be dissolved from the solid phase of cement paste to produce a gel. Most
researchers do
not mention the distinction between “safe” and “swelling” gel but there are
acknowledgments that there are more than one composition of gel produced by
ASR.
The formation of the gel per se is not deleterious. The deterioration of the
concrete structure is due to the water absorption by the gel and its
expansion. The RH must be higher than 80% for the gel to swell although it can be
formed at
lower relative humidity. According to Hobbs the progression of the
swelling of ASR
gel follows the general patterns. As the tensile strength
of the system is
exceeded, cracks will form and propagate. As there is not a preferential
direction for
cracks to propagate and also the sites of crack initiation are randomly
distributed in the
specimen, map cracking will be characteristic of ASR deterioratio. The sites of
the cracks are determined by the location of the reacting silica on the
aggregates and the
availability of OH in the vicinity. |
Aggregates exhibiting this type of reactivity contain various forms of
reactive silica. For convenience, CSA Standard A23.1-Appendix B, divides
alkali-silica reaction into two categories according to the type of reactive
silica involved.
1. Alkali-silica reaction that occurs with poorly crystalline or
metastable silica minerals and volcanic or artificial glasses: Aggregates
containing such materials (see
Table 5-7 and CSA A23.1-Appendix B) may cause deterioration of concrete
when the reactive component is present in amounts as small as 1%. Cracking
of concrete containing these aggregates and a high alkali content is usually
seen within 10 years of construction.
2. Alkali-silica reaction that occurs with various varieties of quartz such
as chalcedony, cryptocrystalline, and macrogranular quartz: Aggregates
containing such forms of quartz may cause deterioration of the concrete when
the reactive component is present in amounts as small as 5% by mass of the
aggregate and there is a high alkali content. Cracking of the concrete may
be seen within 10 years of construction. Canadian experience has been that
this category also includes several slowly expanding aggregates in which
micro-crystalline quartz is thought to be the reactive component. Rocks such
as greywacke, argillite, quartz-wacke, quartzite, hornfels, granite and
granite gneiss are some. See
Table 5-7 and CSA A23.1-Appendix B for a more complete list. Such rock
types may not show cracking and deterioration for up to 20 years. In other
cases however, particularly when exposed to deicing salts, cracking may
occur in 5 years or less.
Visual Symptoms of Expansive ASR. Typical indicators of ASR might be any
of the following: a network of cracks ( Fig.5-20);
closed or spalled joints; relative displacements of different parts of a
structure; or fragments breaking out of the surface of the concrete
(popouts) ( Fig.
5-21). Because ASR deterioration is slow, the risk of catastrophic
failure is low. However, ASR can cause serviceability problems and can
exacerbate other deterioration mechanisms such as those that occur in frost,
deicer, or sulphate exposures.
Mechanism of ASR. The alkali-silica reaction forms a gel that swells as it
draws water from the surrounding cement paste. Reaction products from ASR
have a great affinity for moisture. In absorbing water, these gels can
induce pressure, expansion, and cracking of the aggregate and surrounding
paste. The reaction can be visualized as a two-step process:
1. Alkali hydroxide + reactive silica gel -> reaction product (alkali-silica
gel)
2. Gel reaction product + moisture -> expansion
The amount of gel formed in the concrete depends on the amount and type of
silica and alkali hydroxide concentration. The presence of gel does not
always coincide with distress, and thus, gel presence does not necessarily
indicate destructive ASR.
Factors Affecting ASR. For alkali-silica reaction to occur, the following
three conditions must be present:
1. reactive forms of silica in the aggregate,
2. high-alkali (pH) pore solution, and
3. sufficient moisture.
If one of these conditions is absent, ASR cannot occur. |
Examples of reactive aggregates are chalcedony, porous flint and some
types of sandstone. The reaction leads to the formation of a gel that has a
tendency to absorb increasing amounts of water, which causes swelling. The
swelling forces the concrete to expand, resulting in gel surfacing and loss
of concrete integrity. |